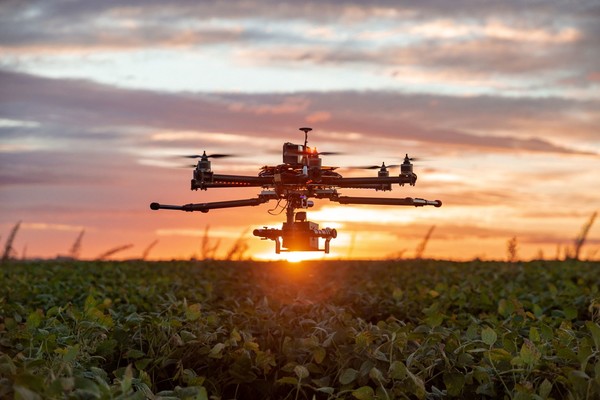
Agricultural drones for sustainability
P |
recision agriculture employs technologies capable of acquiring geo-referenced information from different platforms to obtain a multiplicity of data that will be processed and used for site-specific agroecosystem management. Acquisition platforms can detect information about spatial and temporal variability and are classified according to the distance they hold from the target object into remote platforms, proximal platforms, and ground platforms. There are profound differences among them in terms of cost, usability, practicality, and operating techniques. Among remote platforms are a wide range of monitoring tools used in agriculture, such as satellites, aircraft and drones, which differ deeply in their modes of operation. This is what results in their varied applications in agriculture. In the past decade, to overcome some of the limitations associated with the use of satellites and aircraft, such as low versatility and reduced spatial resolution, Remotely Piloted Aircraft Systems (SAPRs), also referred to as Unmanned Aerial Vehicles (UAVs) or Unmanned Aerial Systems (UAS) or, more commonly, drones, have become increasingly popular and used.
Such platforms have many advantages because of their broad operational flexibility. As a matter of fact, they can be equipped with different types of sensors that make them suitable for a multitude of surveys. Their most noticeable benefits are related to the data acquisition speed as well as the ability to fly below 120 m, a factor that allows for high geometric resolution and, consequently, very detailed information. Recent innovations concerning such platforms have significantly reduced their purchase and operating costs.
Moreover, the temporal resolution allows surveys to be carried out at well-determined times related to agronomic conditions, again depending on the present weather conditions. UAVs face some technical limitations, such as battery efficiency, communication distance and payload. There are different models on the market that can be classified according to the flight mode adopted (Figure 2). They can be divided into two macro-categories, namely fixed-wing and rotary-wing.
Fixed-wing UAVs take advantage of aerodynamic thrust and lift force and are mainly used for spraying and photography over a wide range in orographically homogeneous areas. The macro category of rotary-wing UAVs can be divided into the helicopter and multirotor types.
The helicopter type is characterized by a large propeller on top of the aircraft; multirotor models are characterized by multiple rotors enabling their movement. Based on the different number of rotors, they are divided into quadricopters, hexacopters, and octocopters.
These categories of drones are widely used in Italy because of their versatility of use in fragmented areas with irregular orography.
This is due to the vertical landing and takeoff mode and an easy possibility of altitude variation according to the terrain profile.
There are numerous flight variables that can affect the final acquisition of the data and the entire processing process. Among those that the operator can modify are flight altitude, forward speed, type of flight (manual or automatic), direction of flight, gimbal tilt, frontal and lateral image overlap, lens opening and closing, and focal length. On the other hand, the most critical environmental variables include wind speed and light conditions.
The use of UAV platforms is legally regulated; as of December 31, 2020, the Implementing Regulation (EU) no 947/2019 on standards and procedures for the operation of unmanned aircraft. The Civil Aviation Authority (ENAC), on January 4, 2021, published the UAS-IT Regulations, applicable from December 31, 2020, which regulate the Member States' responsibility. According to the regulations above, a special license is needed in agriculture for the use of such platforms, called A1-A3, which allows the operation of a UAS of operational mass at takeoff between 250 g and 25 kg, under VLOS (Visual Line of Sight) conditions, in areas where reasonably no people are involved and at least 150 meters from residential, commercial, industrial or recreational areas. At the manufacturing level, the main components characterizing UAVs are Chassis, propellers, brushless motors, central flight management unit (control unit or Flight Control), ECS (Electronic Speed Control), IMU (Inertial Measurement Unit) systems which include accelerometers, gyroscopes, compass, magnetometer and altimeter, the PMU (Power Management Unit), the flight management system (Navi Control), a GNSS (Global Navigation Satellite System) module, the communication systems (usually radio) between the platform and the radio control, and finally the payload consisting of gimbals and sensors.
As drones are equipped with a GNSS system, they can capture geo-referenced frames containing geometric, spectral and thermal information through various sensors. Such sensors can be CMOS (complementary metal oxide semiconductor) or CCD (charge-coupled device) and affect the final pixel size and the effect of noise in images. Depending on their construction technology, sensors are classified into active and/or passive depending on whether or not they use external illumination sources.
In precision agriculture, the main types of sensors used for monitoring activities from UAVs are RGB, multispectral, hyperspectral, thermal, and LiDAR chambers. RGB chambers are mainly used for topographic surveys of the territory, monitoring of vegetation growth, and acquiring biophysical information about the canopy (diameter, area and volume; Fig. 4); it is also possible to detect the evolution of phenological stages and quantify the yield of certain crops.
They can also be used to estimate the damage to crops due to flooding from river overflow (Fig. 5). Multispectral sensors generally use a few bands (3-10) with a high amplitude (15-70 nm) and provide quantification of the eco-physiological state of crops through the calculation of vegetation indices, such as the normalized vegetation index (NDVI).
Such indices help to create continuous maps over the entire plot that can describe the spatiotemporal distribution of a given variable, such as the vigor map that allows monitoring the vegetative growth of the crop (Figures 6 and 7).
Other indices enable detailed assessment of certain pigments, such as chlorophyll pigments, to understand the health status or even identify the phytosanitary status of the crop.
Hyperspectral technology captures images capable of capturing a large number of bands (1000-2500) of reduced amplitude (1-5 nm) up to describing the full spectral signature of the target object.
These sensors detect information from special chromophore molecules that identify a particular level of crop stress.
Nonetheless, their use in agriculture is still limited because of their high cost and some compatibility constraints with certain drones.
Thermal sensors, which measure the thermal emission of vegetation, are another type of sensor mainly used for water resource management.
Lastly, LiDAR sensors are one of the most accurate methods for acquiring geometric data.
They allow, in fact, to detect dense point clouds and, thanks to graphical processing performed with special software, can accurately reconstruct the 3D geometry of a crop. These sensors enable canopy volume estimation and reliable crop surface models (CSM – Crop Surface Model). Understanding field-scale variability enables the application of spatially variable inputs (VRA, Variable Rate Application) through the use of prescription maps.
This results in an economic and environmental benefit in crop management compared to uniform plot management. The scale of VRA applicability can vary depending on the agronomic context, managing the entire plot by homogeneous zones, by sectors, by plots, or even by single plants. In precision olive cultivation, VRA application of fertilization leads to significant fertilizer reductions (about 30%), resulting in increased sustainability.
Precise and effective characterization of canopies in tree systems, evaluated using the technologies shown, allows for modification of pesticide application rates in vineyards up to 20-40% savings compared to a conventional system. Therefore, it is clear that UAV systems are a monitoring tool that is well suited for future developments in precision agriculture, whose future directions (DSS, crop development models, etc.) need high-precision, high-accuracy information for better site-specific management.